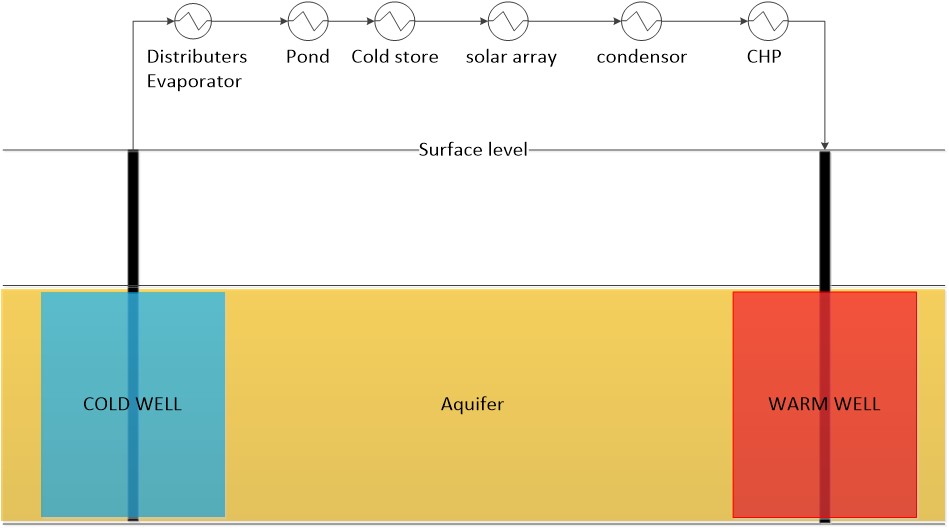
Unique selling points
- Seasonal and/or long-term energy storage of thermal energy
- Subsurface heat storage with long-term heat recovery efficiencies up to 83%
- Subsurface cooling capacity with long-term cooling efficiencies up to 93%
Description of the technology
In an aquifer thermal energy storage (ATES), excess heat is stored in subsurface aquifers in order to recover the heat at a later stage. The thermal energy is stored as warm groundwater. The groundwater is also used as a carrier to transport the heat to and from the subsurface. Hence, the thermal energy is stored and recovered via the production and injection of groundwater from an aquifer through wells. The capacities of ATES systems range from 0.33 MW to 20 MW (Fleuchaus et al. 2018).
Usually, the ATES is operated seasonally. In summer, the excess heat from gas or coal fired power plants, from solar plants or from cogeneration plants is transferred via heat exchangers to the cold groundwater. The resulting warm groundwater transports the heat into the aquifer, where the heat is stored. In winter, the ATES is operated the opposite direction by reversing the flow in the production and injection wells. Now, the stored heat is recovered from the warm groundwater via heat exchangers and used for heating purposes, while the resulting cold groundwater is reinjected in the aquifer.
Usually, the distance between the injection and production wells is between 1000 m and 2000 m (Stober and Bucher 2014). The depth of the aquifer also varies. In Berlin for example the depth of the ATES is between 30 m and 60 m in a shallow aquifer, while in Neuruppin it is at around 1700 m. In the Netherlands, most of the ATES systems use aquifers in depths between 20 m and 150 m in the subsurface (Bloemendal and Hartog 2018).
Corresponding to the depth, heat storages are operated at different temperatures. Low temperature (LT) ATES are operated below 30°C and are usually located in shallow aquifers, medium temperature (MT) ATES refer to a temperature range between 30 °C and 50°C and high temperature (HT) ATES are operated at 50°C and higher (Lee 2013).
In contrast to MT- and HT-ATES, due to the low temperature in LT-ATES, a heat pump is used to increase the temperature to the level required to heat the associated building such as 40 °C. Simultaneously, the extracted groundwater is cooled to a temperature between 5 °C and 8 °C. Subsequently, the cold groundwater is reinjected in the cold well. In summer, buildings can be efficiently cooled using groundwater from the cold well. This water is heated due to the cooling process by the heat pump to a temperature range between 14 °C and 18 °C. Subsequently, the heated groundwater is stored via the warm well in the LT-ATES for its later recovery in winter. If the cooling requires no facilities next to the low-temperature groundwater stored in the previous winter season, it is called free cooling. When excess heat is available, e.g. from solar collectors, or waste heat, this can be added to the warm well to reduce heat pump operation or make it even redundant.
In the NextGen project, by the MT-ATES system, the excess of heat from solar collectors, CHP, lighting, cold store and surface water is stored in an aquifer (depth between 50 m to 180 m below surface) so that it can be recovered in winter period for heating purposes. Figure 3 shows some images of the inside of the plant.
In the ULTIMATE project, the feasibility for the application of a HT-ATES system to store excess heat from geothermal systems was studied. By storing the produced heat in summer and delivering this in winter during peak demands, the utilization of the geothermal heat and the reduction of emissions increase. Hence, the combination of a geothermal system and HT-ATES allows for optimal utilization of the available heat. For geothermal systems (and several other sources of excess heat) a HT-ATES system is required (Drijver et al. 2019; Kallesøe and Vangkilde-Pedersen 2019). The technical and economical feasibility showed that the use of HT-ATES results in an increase of 6%-points of the utilisation of the heat available from the geothermal wells, in particular from 92% yield in the situation without HT-ATES to 96-98%.
Flow scheme of the technology
Fig. 1 General scheme of an HT-ATES
Fig. 2 Scheme of an LT-ATES as in NextGen (summer mode)
Pictures
Synergetic effects and motivation for the implementation of the technology
- Excess heat can be stored over a long time period e.g. a season or even longer
An ATES stores the heat over a long time period ranging between several months up to several years. The heat can be recovered, whenever it is needed.
- High storage capacity
Due to the high storage mass of an ATES, its storage capacity is high compared to other thermal storages.
- Heating and cooling dependent on the season
Simultaneously to the storage of waste heat from industrial processes and its recovery for heating purposes, also, the cold groundwater can be used for cooling processes in the summer. Thus, it can replace for example conventional air conditioning and heating units.
- Reduction of CO2 emissions
The ATES system allows to store and recover waste heat or to provide cool groundwater for cooling purposes. This significantly reduces the amount of fossil fuels needed for heating in winter or for cooling in summer.
- Sewer heat recovery
Temperature levels in LT-ATES are ideally matching with sewer heat recovery. Therefore, LT-ATES is a perfect match for decoupling heat availability and heat demand in sewer heat recovery systems.
Technology requirements and operating conditions
The thermal storage potential of an ATES depends on the mineralogical composition of the rocks in the aquifer, the compaction, stratification, porosity and permeability of the aquifer. Furthermore, waterlogged areas of an ATES have a higher thermal storage potential due to their higher heat capacity and their higher thermal conductivity compared to areas containing air or gases (Tab.1).
Tab. 1 Parameters influencing the thermal storage potential of an aquifer (Stober and Bucher 2014, Freeze and Cherry 1979)
Parameter |
Units |
Sandstone |
Water bearing sand/gravel |
Dry sand/gravel |
Specific heat capacity |
kJ/(kg*K) |
0.82-1.00 |
0.85-1.90 |
0.50-0.59 |
Vol. heat capacity |
MJ/(m³*K) |
1.6-2.8 |
2.3-3.0 |
1.4-1.6 |
Thermal conductivity |
J/(s*m*K) |
1.3-5.1 |
1.7-5.0 |
0.3-0.8 |
Porosity |
% |
30 |
> 40 |
|
Permeability |
cm² |
10-13 – 10-9 |
10-9 – 10-3 |
|
Hydraulic conductivity |
m/s |
10-10 – 10-6 |
10-6 – 1 |
In the ULTIMATE case study “Nieuw Prinsenland”, a combination of method for logging and screening of possible suitable layers, while drilling of a geothermal well, was developed (Bloemendal and Beernink, 2022). The approach consisted of deepening the conductor drilling of the geothermal well. Taking cutting samples, core samples and the use of geophysical logging with different logging probes using the following sensors: gamma ray, Short Normal and Long Normal electrical resistance measurement, Single Point Resistance, Electro Magnetic Induction and spectral Gamma Ray. It was demonstrated that this approach allows cost effective data acquisition in shallow layers during drilling activities for geothermal systems.
In Tab. 2, examples for HT-ATES and LT-ATES are presented with their characteristics and operating conditions. The ATES in Neuruppin, Neubrandenburg and Berlin are located in north Germany, while Westland is in the Netherlands. For the LT-ATES in Berlin, two aquifers in different depths are used. For the cold store, the upper aquifer at a depth of around 60 m is used and for the heat store, the aquifer in a depth of around 320 m is used (Sanner et al. 2005). Tab. 2 shows the operating conditions for the cold store.
Tab. 2 Characteristics and operating conditions of HT-ATES and LT-ATES (n.i. = not indicated)
Parameter |
Units |
Example Neuruppin (HT-ATES) |
Example Neubrandenburg (HT-ATES) |
Example Berlin (LT-ATES; cold store) |
NextGen Netherlands (MT-ATES) |
Reservoir rock |
- |
Sandstone |
Sandstone |
Sand |
Sand |
Depth |
m |
1700 |
1250 |
30-60 |
50-180 |
Tcold/Twarm |
°C |
50/63 |
45-54/65-85 |
6/30 |
5/30-45 |
Injection flow rate (max) |
m³/h |
50 |
80 |
300 |
160 |
Literature |
- |
Key performance indicators
Via energy and exergy analyses, the heat stored and recovered can be determined. Thereby, the thermal storage potential of an ATES depends on the mineralogical composition of the geological formation of the aquifer, its compaction, stratification and permeability. Furthermore, also the characteristics of the geothermal fluid such as its temperature, salinity and the dissolved gases influence the efficiency of the heat transfer from and to the fluid and the heat transport. The recovery factor is a typical key performance indicator showing the recovery efficiency of warm or cold stored thermal energy over the whole charge and discharge cycle (normally referred to one year). The recovery factor is defined by the ratio between the annually discharged and charged energy. Here, the operational age of an ATES plays a crucial role. According to Todorov et al. 2020, after 3 to 4 years of operation, the HRF converges. Tab. 3 shows typical values for warm and cold thermal energy recovery.
Tab. 3 Recovery factors for warm and cold thermal energy (n.i. = not indicated)
Parameter |
Units |
Min |
Max |
References |
Recovery factor for warm thermal energy |
- |
0.57 |
0.89 |
Paci 2016, Todorov et al. 2020; Holstenkamp et al. 2017, Bloemendal et al. 2020 |
Recovery factor for cold thermal energy |
- |
n.i. |
0.93 |
In the following table, the charged and discharged energy are shown. In Neubrandenburg the HT-ATES is operated since 1987 and the LT-ATES in Berlin since 2003.
Tab. 4 Examples for annual energy charge and discharges (n.i. = not indicated)
Parameter |
Units |
Neubrand-enburg HT-ATES |
Berlin LT-ATES (heat store) |
Berlin LT-ATES (cold store) |
Westland (NL) MT-ATES |
Start of operation |
- |
1987 |
2003 |
2003 |
2015 |
Energy charge |
MWh/a |
12,000 |
2,650 |
4,250 |
4,200 |
Energy discharge |
MWh/a |
8,600 |
2,050 |
3,950 |
3,750 |
Recovery factor |
- |
0.72 |
0.77 |
0.93 |
0.89 |
Reference |
|
Links to related topics and similar reference projects
Processes/ technologies |
Reference |
ATES |
|
HT-ATES |
|
Case Studies applying the technology
Publications
- Bloemendal, M., Beernink, S., HT-ATES feasibility for TRIAS Westland, 2023
- Bloemendal, M., Beernink, S., Bel, N. van, Hockin, A., Schout, G., Open bodemenergiesysteem Koppert-Cress met verhoogde opslagtemperatuur, 2020
- Bloemendal, M., Hartog, N., Analysis of the impact of storage conditions on the thermal recovery efficiency of low-temperature ATES systems, 2018
- Drijver, B., Bakema, G., Oerlemans, P., State of the art of HT-ATES in The Netherlands, 2019
- Fleuchaus, P., Godschalk, B., Stober, I., Blum, P., Worldwide application of aquifer thermal energy storage – A review, 2018
- Freeze, R., Cherry, J., Groundwater, 1979
- Holstenkamp, L., Meisel, M., Neidig, P., Opel, O., Steffahn, J., Strodel, N., Lauer, J., Vogel, M., Degenhart, H., Michalzik, D., Schomerus, T., Schönebeck, J., Növig, T, Interdisciplinary review of medium-deep aquifer thermal energy storage in North Germany, 2017
- Kallesøe, A., Vangkilde-Pedersen, T., Underground Thermal Energy Storage (UTES) – state-of-the-art, example cases and lessons learned, 2019
- Kim, J., Hofman, J., Kenyeres, I., Poor-Pocsi, E., Kleyböcker, A., Heinze, J., Kraus, F., Soares, A., Paissoni, E., Vale, P., Palmer, M., Bloemendal, M., Beernink, S., Ros, S., Hartog, N., Frijns, J., D1.4 (2022) New approaches and best practices for closing the energy cycle in the water sector, 2022
- Lee, K., Underground Thermal Energy Storage, 2013
- Lerm, S., Mikroorganismen in geothermischen Aquiferen – Einfluss mikrobieller Prozesse auf den Anlagenbetrieb, 2012
- Lerm, S., Alawi, M., Miethling-Graff, R., Wolfgramm, M., Rauppach, K., Seibt, A., Würdemann, H., Influence of microbial processes on the operation of a cold store in a shallow aquifer: impact on well injectivity and filter lifetime, 2011
- Paci, L., Pasquinelli, L., Fabricius L., Overview of high temperature aquifer thermal energy storage (HT-ATES): Challenges and strengths, 2016
- Sanner, B., Kabus, F., Seibt, P., Bartels, J., Underground thermal energy storage for the German parliament in Berlin, system concept and operational experiences, 2005
- Stober, I., Bucher, K., Geothermie, 2014
- Todorov, O., Alanne, K., Virtanen, M., Kosonen, R., A method and analysis of aquifer thermal energy storage (ATES) system for direct heating and cooling: A case study in Finland, 2020